<< Back to MOTIFvations Blog Home Page
Single-Cell DNA Methylation Sequencing: Small Scale Differences Explain Big Effects!
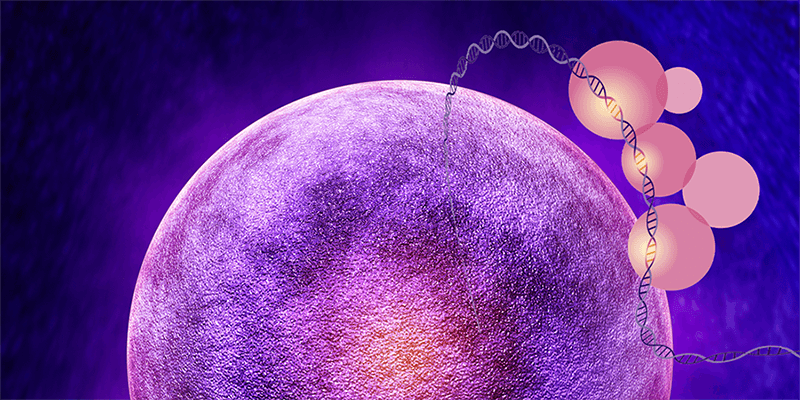
By Michelle Tetreault Carlson, Ph.D.
October 13, 2022
Table of Contents:
Introduction to Single-Cell Sequencing
Traditional bulk sequencing methods analyze the genome, transcriptome, or epigenome from a homogenized collection of cells originating from a piece of tissue or cell culture, giving us an average picture of each of these particular “ome” states. However, cell populations are heterogeneous. One tumor can contain different zones of cell morphology, genomic mutations, and gene expression. Brain circuits contain multiple types of neurons, each with their own molecular, connectional, and functional characteristics. Even microbes cultured from a single clone in a well-mixed suspension exhibit individual differences in how they grow and reproduce. To understand these variations, there has been a rapid development of technologies in recent years, which allow us to assay the states of individual cells, referred to as single-cell sequencing or single-cell omics.
Single-cell RNA sequencing (scRNA-Seq) has advanced rapidly ever since it was first performed on 4-cell stage mouse blastomeres, 2009 by Tang et. al. Since then, scRNA-Seq has matured, becoming more consistent, reproducible, and high throughput. scRNA-Seq reveals the variability in RNA transcripts in populations of cells. It’s been applied to the study of typical embryonic and immune cell development, as well as to look at diseased cells and progression. Single-cell genome sequencing (scDNA-Seq) has also been developing steadily, although a bit more technically challenging, as amplifying and sequencing large regions of DNA lends itself to amplification bias, genome loss, and false mutations. A number of clever techniques have been developed to address these issues, which now make it possible to determine single nucleotide variants (SNVs) and copy number aberrations (CNAs) in single cells. Single-cell DNA sequencing has shed light on biological questions that were previously impenetrable across many research fields, including somatic mutagenesis, organismal development, genome function, and microbiology.
More recently, a whole slew of new single-cell sequencing technologies has emerged which apply to the epigenome. These have extended our understanding of single cell behavior to DNA accessibility, chromosome conformation, and methylation. Single-cell ATAC-Seq (scATAC-Seq), first introduced the Greenleaf and Shendure labs in 2015, and discussed in a previous Active Motif blog, is used to determine chromatin accessibility in individual cells. Single-cell ChIP-Seq (scChIP-Seq), also introduced in 2015, is being used to map the chromatin state at single-cell level. Single-cell Hi-C, introduced in 2013, is being used to ascertain the cell-to-cell variability in the 3-D spatial organization of chromosomal structure.
Here we aim to focus on the status of methods being used to map DNA methylation at a single-cell level; covering the common difficulties encountered, how they are addressed, and how these techniques are being applied to better understand the cell heterogeneity involved in cell development, disease, and brain structure.
Methods for Mapping Single-Cell DNA Methylation
Traditional techniques to study the DNA methylome require substantial amounts of DNA. Bisulfite conversion, still the gold standard for assaying methylation at single nucleotide resolution, causes degradation of DNA samples. And as one might imagine, this is especially problematic when trying to determine methylation at a single-cell level, where any loss of sample is critical. While a few less harsh, enzymatic conversion methods have been introduced more recently, such as TET assisted pyridine borane sequencing (TAPS) and enzymatic methylation-Sequencing (EM-Seq), at this time neither is used for single-cell sequencing. Only bisulfite conversion-based approaches have been used for single-cell methylation sequencing. The first is whole genome bisulfite sequencing (WGBS), where the entire genome is profiled. The second is reduced representation bisulfite sequencing (RRBS) where regions of high CpG content (CpG islands) are enriched for and sequenced. Both methods involve bisulfite conversion and library preparation for NGS sequencing. To address issues such as sample loss in bisulfite-based sequencing, some clever strategies have been applied to make single-cell DNA methylation mapping possible.
RRBS-Based Single-Cell Methods
RRBS is a bisulfite-based method which enriches regions of high CpG methylation so that many fewer sequencing reads are required. In conventional RRBS, restriction enzymes are first used to digest DNA adjacent to targeted CG sites. Sequencing adapters are then ligated, followed by size selection, bisulfite conversion of the DNA, and amplification. Between all these steps DNA purification is normally needed, with each purification step resulting in sample loss. If starting with single cells, there is no guarantee that one’s region of interest will ever make it to the final amplification process.
One approach to minimize this loss is to integrate several of the required steps into a single-tube reaction. Reagents can sequentially be added to the cells or nuclei in a tube, without purification steps in between. By reducing transfer of DNA and purification, a substantial source of loss can be avoided. Another tactic is to add a carrier like tRNA. Small amounts of DNA stick to the surfaces of materials like plastics which are used for DNA manipulation. If working with just small amounts of DNA to begin with, this can be a large percentage of the sample. Carriers saturate these surfaces so that the DNA sample won’t stick and get lost.
Microfluidic devices are also being used to reduce sample loss in scRRBS-Seq. Microfluidic systems are highly miniaturized devices which use chambers and tunnels to control the flow of liquids in and out; ideal for the manipulation of single cells. In microfluidic diffusion based RRBS (MID-RRBS), microfluidics is used to profile single cells. This method uses a diffusion-based reagent swapping approach for multi-step treatment of DNA on the microfluidic system. Reagent exchange can occur with minimal loss of DNA during purification as well. The protocol preserves substantially more amplifiable DNA than conventional bisulfite treatment while achieving a high conversion rate.
In addition to sample loss, amplification bias is a problem for single-cell methods. Tiny amounts of DNA require many rounds of PCR amplification before sequencing. To address this, unique molecular identifiers or UMIs are commonly used for scRRBS. UMIs are short molecular codes used to tag each individual cell, so that differences in PCR amplification in distinct fragments of DNA can be revealed. In a method referred to as Q-RRBS UMIs are used so duplication-induced artifacts can be quantified. In addition, Q-RRBS can accurately detect allele-specific methylation in the absence of allele-specific genetic variants.
WBGS-Based Single-Cell Methods
Even though more expensive, WGBS, which gives genome wide coverage of methylation, is often desired over RRBS, which only covers CpG islands. WBGS is a bit simpler than RRBS in that it doesn’t require the enzymatic digestions, size selection, and all the associated sample sapping purification steps. In traditional bisulfite sequencing, DNA is fragmented, adapter ligated, bisulfite treated, and then PCR amplified. So, sample loss in WGBS is mainly due to the bisulfite conversion.
Because bisulfite treatment occurs after adapter ligation, many fragments which contain intact sequencing adapters at each end, are degraded, becoming unsequenceable. In 2012, a method known as post-bisulfite adaptor tagging (PBAT) was developed for bisulfite sequencing. With PBAT, bisulfite treatment is done immediately after fragmentation of the DNA and before adapter ligation. This is made possible by using a primer for first strand synthesis which contains a random tetramer at one end and a biotin tag at the other. Strands are immobilized on streptavidin-coated magnetic beads so that a second strand can be synthesized using a second primer containing a random tetramer. As a result, a sizeable number of unamplified reads can be created from sub nanogram quantities of DNA.
Many other strategies (each with their own unique names) have since been added to the original PBAT approach to improve upon it for single-cell methylome profiling. With single-cell bisulfite sequencing (scBS-Seq) bisulfite conversion is done in the cell lysate and the primers contain a random hexamer instead of a random tetramer. Original PBAT did not do any amplification. In scBS-Seq, five cycles of amplification on the bisulfite-converted DNA are done during first strand synthesis. Additionally, ~10-15 cycles of amplification are performed using adaptor sequences after second strand synthesis. To reduce sample loss, purification with streptavidin beads is replaced with solid-phase reversible immobilization (SPRI) beads.
In another method dubbed scWGBS, instead of performing multiple cycles during first strand synthesis as in scBS-Seq, terminal tagging is done during first strand synthesis. Up to 18 cycles of amplification are then performed during second strand synthesis using the tagged sequence. In snmC-Seq and in the improved snmC-Seq2, nuclei are first isolated instead of cell lysate. And by using the enzyme Adaptase™, which enables the addition of an adapter onto single-stranded DNA, single-strand NGS preparation was made possible. This is not a complete list of advances and methods. However, it’s fair to say that many incremental changes have been and continue to be made to improve the process.
Applications of Single-Cell DNA Methylome Studies
DNA methylation is critical for numerous cellular functions including gene expression, embryonic development, genetic imprinting, and X chromosome inactivation. By applying DNA methylome analysis to single cells, we can gain a cell type-specific glimpse into how epigenetics is involved in both normal cell development and disease.
Cell Development Studies
The normal DNA methylation patterns which constrain aberrant gene expression in somatic cells, are remodeled during the development of germ cells and immediately after fertilization in pre-implantation embryos. At these times DNA methylation must be reversed, so that the cell differentiation required for the creation of a new human can occur. Exactly how this happens, and the timing of the DNA methylation involved is of great interest. But up until recently the single-cell techniques required to monitor such a small number of cells were not possible. Now, answers to some of these questions are being uncovered.
In a paper by Yu et. al., scWGBS was used to look at DNA methylation patterns of developing human oocytes. Human oocytes undergo several stages of maturation during each menstrual cycle. Each month, 10-15 germinal vesicle oocytes develop but only 1-2 reach maturity before ovulation. The rest of them degenerate. The genetic and epigenetic basis for this selection process is not well understood. In this work, both CpG and non-CpG methylation levels were examined throughout different oocyte maturation stages. It was found that while broad-scale patterns of CpG methylation are largely established by the germinal vesicle stage, localized changes continue into later development. Non-CpG methylation, on the other hand, undergoes a large-scale, generalized remodeling through the final stage of maturation, with the net overall result being the accumulation of methylation as oocytes mature.
In other work published by published by Sen et. al., a cost-effect method they call scMSPJI-Seq was used to quantify strand-specific 5mC methylation in DNA in single cells. Strand specificity was used to distinguish between passive and active demethylation occurring in early-stage mammalian development. Passive demethylation occurs during successive rounds of cell division, in the absence of DNA methyltransferases available to restore methylation on the newly formed complementary strand. With passive demethylation, the result is asymmetric levels of 5mC on chromosomal DNA strands. In active demethylation, 5mC is actively converted to 5hmC via enzymes and is not restored by the normal DNA maintenance machinery so that methylation is removed from both strands. Both mouse embryonic stem cells and pre-implantation embryos were employed in these experiments. It was found that in mouse embryonic stem cells, there was great cell-to-cell variability in strand-specific methylation. The pre-implantation embryos underwent two distinct phases of methylation dynamics, with an initial phase of active methylation maintenance up until the 16-cell stage, followed by loss of that maintenance in a fraction of the cells at the 32-cell stage.
Disease-Associated Studies
Multiple epigenetic systems including DNA methylation function cooperatively and independently to establish the epigenome that propagates healthy cell gene expression profiles. Disruption of these epigenetic mechanisms can lead to disease. Many researchers are now incorporating single-cell DNA methylation profiling into their studies of these abnormal systems to understand its involvement.
Tumor heterogeneity is a recognized phenomenon that can make treatment difficult as it contributes to therapeutic resistance. In a research study published by Johnson et. al. single-cell reduced representation bisulfite sequencing (scRRBS-Seq) and single-cell RNA sequencing (scRNA-Seq) were performed on single cells separated and sorted from 11 patient gliomas. Gliomas are a type of incurable malignant brain tumor originating in glial cells and which exhibit considerable heterogeneity. In addition to single-cell sequencing, bulk genomic, bisulfite, and RNA sequencing were done on the same tumor samples to look for any association between epigenetic heterogeneity, genetic alterations, cellular states, and glioma stress response. It was found that early genetic alterations were associated with DNA methylation disorder and that accumulation of this disorder correlated with both cellular states and response to environmental stress such as hypoxia and irradiation. Increased DNA methylation disorder was also associated with accelerated disease progression. This work identified an epigenetically driven adaptive stress response in glioma tumors which underscores the importance of looking at epigenetic heterogeneity in therapeutic outcomes.
In another study by Ubrva et. al. a single-cell multi-omics approach was used which integrated DNA methylation (scBS-Seq), chromatin accessibility (scATAC-Seq), and transcriptomics (scRNA-Seq) to better understand the causes behind common variable immunodeficiency (CVID). CVID is an immune system disorder which results in low serum antibody concentrations. Symptoms of the disease include repeated infections of the respiratory and gastrointestinal system. There is also an increased risk of autoimmune disease and cancer. For most patients there is no family history, and the severity of symptoms can vary greatly from person to person. A previous study done with a set of identical twins, one with and one without CVID, suggested that alterations in DNA methylation were associated with the impairment of memory B cell development in the twin with CVID. In this study by Ubrva et. al., the association was investigated further, using a large cohort of patients with and without CVID, and a single cell multiomics approach to better understand the possible role of epigenetic dysregulation in CVID. PBMCs were collected, sorted, and libraries generated using single-cell bisulfite sequence (scBS-Seq). The data indicated widespread alterations in DNA methylation and chromatin accessibility in memory B cells and provided evidence that these changes impact the transcriptome of these cells following activation.
Brain Studies
Mammalian brain cells differ vastly in their gene expression, morphology, and function. Up until recent advances in single-cell analysis technology, the underlying mechanisms which control this heterogeneity have been difficult to sort out. In work done by Hanqing Liu et. al. the single-cell DNA methylation sequencing technique scmC-Seq2 was used to create a DNA methylation atlas of mouse brain cells. ScmC-Seq2 data was integrated with single nucleus ATAC-Seq chromatin accessibility data to predict enhancer-gene interactions in these cells. More than 100k nuclei from broad regions of the mouse brain were profiled at single cell resolution, including neuronal and non-neuronal cells. They were able to identify 161 different mouse brain cell subtypes, each with its own unique CpG methylation pattern. More specifically, differentially methylated transcription factor genes and binding motifs were associated with taxonomy branch subtypes, enabling the prediction of cell-type specific gene regulation for each developmental lineage. DNA methylation was shown to be the epigenetic basis for neuronal diversity and spatial organization. This technique proves to be a valuable method for answering fundamental questions about how cell specific epigenomics controls gene regulation.
Summary
As an epigenetic mark, DNA methylation reveals valuable information on what’s going on inside a cell. In traditional bulk sequencing methods, DNA methylation signals are averaged out so that cell to cell variability in methylation is lost. Single-cell methods for DNA methylation can reveal these hidden signatures. But gathering DNA methylation data on a single-cell level has been challenging because of damage done by bisulfite conversion, still considered the gold-standard method for determining methylation at single base resolution. To overcome this disadvantage many clever tactics, such as PBAT and microfluidics are being applied to RRBS and WGBS to minimize DNA loss during single-cell DNA methylation prep. These methods are uncovering valuable insights into cell development, disease, and brain cell heterogeneity. As these have only been around for a short number of years, it’s almost certain that we’ve only just scratched the surface.
Key References:
Ahn J, Heo S, Lee J, Bang D. Introduction to Single-Cell DNA Methylation Profiling Methods. Biomolecules. 2021 Jul 10;11(7):1013. doi: 10.3390/biom11071013. PMID: 34356635; PMCID: PMC8301785.
Sun R, Zhu P. Advances in measuring DNA methylation. Blood Sci. 2021 Dec 6;4(1):8-15. doi: 10.1097/BS9.0000000000000098. PMID: 35399541; PMCID: PMC8975094.
Kashima Y, Sakamoto Y, Kaneko K, Seki M, Suzuki Y, Suzuki A. Single-cell sequencing techniques from individual to multiomics analyses. Exp Mol Med. 2020 Sep;52(9):1419-1427. doi: 10.1038/s12276-020-00499-2. Epub 2020 Sep 15. PMID: 32929221; PMCID: PMC8080663.
About the author
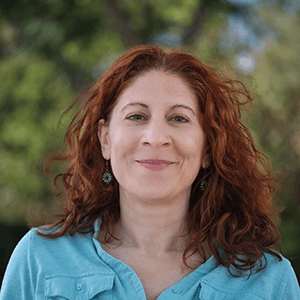
Michelle Tetreault Carlson, Ph.D.
Michelle’s interest in science was first spurred by the starry skies above her rural farm in upstate New York State, leading her to pursue a B.S. in physics. She was originally interested in astrophysics when entering the University of California, San Diego, but transitioned towards the more practical pursuit of biology earning her Ph.D. in Biophysics, studying photosynthetic proteins. Michelle’s postdoctoral research on retinal ion channels, took her further towards biology, ultimately leading to a career in the biotech industry. She enjoys chatting with scientists about their projects and interacts with them both as a Technical Support Scientist and Product Manager for Active Motif’s DNA Methylation products.
Michelle is a mother of 4 kids and 2 cats, and her hobbies include puzzles (the sign of a patient and logical mind), cooking, and pondering the human condition.
Contact Michelle with any questions at [email protected]
Related Articles
Complete Guide to Understanding Single‑Cell RNA‑Seq
March 4, 2021
Single-cell RNA-seq techniques have made it possible to study transcriptomics in heterogeneous samples; driving advances in our understanding of cancer, embryonic development and neurodegenerative disease. This article covers the history, protocols, and applications of Single-cell RNA-seq.
Read More
ABBS – A Novel Method to Map DNA Methylation Genome-Wide at Base Resolution
July 11, 2022
Anchor-based bisulfite sequencing (ABBS) was developed for detection of DNA methylation genome-wide. ABBS is a new technology developed by Active Motif and recently described in Communications Biology (Chapin et. al., Commun Biol., 2022).
Read More
Beginner’s Guide to Understanding Single-Cell ATAC-Seq
September 30, 2020
In this article, we describe how single-cell ATAC-Seq (scATAC-Seq) works and highlight the benefits and the drawbacks of scATAC-Seq relative to bulk ATAC-Seq.
Read More
<< Back to MOTIFvations Blog Home Page