<< Back to MOTIFvations Blog Home Page
Guide to Understanding the Benefits and Uses of Recombinant Antibodies

By Anne-Sophie Ay-Berthomieu, Ph.D.
May 21, 2020
Antibodies are fascinating proteins. They are produced naturally as part of the immune system response to infection by viruses or bacteria, as well as to protect against the development of other diseases such as cancer. Antibodies also bind to their target with incredibly high specificity and affinity, which led to them being developed as extremely important research tools. This article covers what recombinant antibodies are, and how they are different from polyclonal and monoclonal antibodies.
What are Antibodies?
Antibodies (Abs) are proteins that belong to a family of proteins called immunoglobulins (Igs). Antibodies are produced naturally by lymphocytes, generally in response to infection by microorganisms such as bacteria or viruses, and are integral components of the immune system. The most common type of antibody produced by humans is a type of protein called immunoglobulin G (IgG). The molecules that antibodies recognize and bind to are referred to as antigens. The antibody-antigen interaction is very specific and very strong.
Because of the high affinity and specificity between antibodies and antigens, Abs have also been developed as important tools that are commonly used in research experiments. The first article reporting the use of antibodies in research was published in 1941 by Albert Coons, Hugh Creech, and Norman Jones, from Harvard Medical School and Harvard University. They tested the use of an anti-pneumococci type III rabbit antibody labeled with a fluorescent dye for tissue immunostaining.
Since those early days, the use of antibodies in research has been extended to many different kinds of experiments.
Three main types of antibodies are now being used in research and clinical applications: monoclonals, polyclonals, and recombinant antibodies. Each type of antibody displays different advantages and disadvantages depending on the final application.
In this article, we discuss the history of using antibodies in research and how antibody technology has evolved, including how antibodies have been adapted for diagnostic and therapeutic purposes.
A Brief History of Using Antibodies in Research
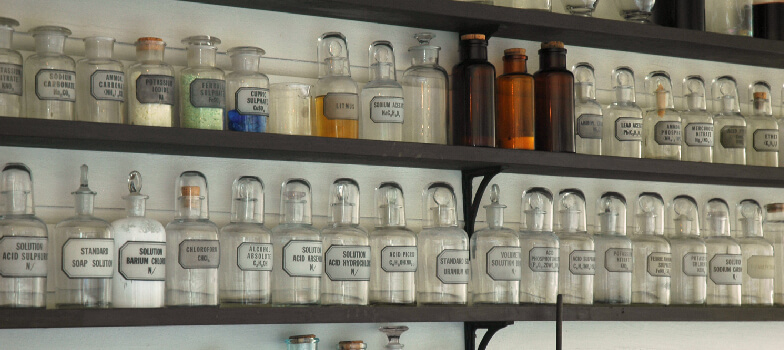
The First Steps Taken in Understanding Antibodies
The first research leading to the discovery of antibodies was in 1890 by Emil von Behring and Shibasaburo Kitasato. They showed that serum from animals infected with diphtheria can prevent infection and treat other animals. In 1900, Paul Ehrlich, who was in charge of improving and standardizing Behring's antidiphtheritic serum production, proposed the idea that cells have "side chains" that could link to specific toxins. At this time, the notion of what antibodies were started to materialize. Ehrlich was granted the Nobel Prize in Physiology or Medicine in 1908, jointly with Ilya Ilyich Mechnikov, for their work on immunity.
As mentioned previously, the first use of antibodies as an analytical tool was published in 1941 by Albert Coons, Hugh Creech, and Norman Jones from Harvard Medical School and Harvard University. The objective of their research was to develop a method that allowed antigenic substances to be identified and investigated in mammalian tissues. Anti-pneumococci type III rabbit serum was conjugated with a new type of fluorescent compound that the scientists thought would deliver improved sensitivity in their assays over the labels that were previously used at the time. Coons and colleagues found that after labeling the antibodies in their serum and incubating them with pneumococci type II and III, type III bacteria, but not type II, fluoresced after UV exposure. Thus, the fluorescent conjugation was confirmed to not impair antibody function, and the immunofluorescence technique was born.
The second analytical use of antibodies was described by Robin Coombs, Arthur Mourant, and Robert Race from the University of Cambridge (UK) in 1945. This team of researchers developed the Comb test, which is an antiglobulin test that detects anti-Rho (anti-D) non-agglutinant antibodies. The original purpose of this study was to understand the mechanisms underlying the hemolytic disease of newborn babies. This pathology is due to the incompatibility between an Rh-negative mother sensitized during a previous pregnancy with her Rh-positive fetus. The anti-D IgG Ab produced by the mother can cover the fetal red blood cells leading to their phagocytosis. The Comb test was first developed for research and is now widely used for the diagnosis of hemolytic disease.
Major Technical Advancements Using Antibodies in Research
During the 20th century, the use of antibodies was adapted to many different laboratory techniques and the technology to produce antibodies also evolved. The following is a brief list of some of the major discoveries and milestones in the development of antibodies for research use.
- 1949: First Co-IP. Kesztyus L., Nikodemusz S., and Szilagyi, T. demonstrated an interaction between myosin and actin in muscle by co-immunoprecipitation.
- 1960: First Radioimmunoassay. The radioimmunoassay was developed by Rosalyn Yalow and Solomon Berson to quantify insulin levels present in blood plasma. The goal of their study was to measure the level of insulin during a glucose tolerance test and in diabetic people.
- 1970s: First IHC and ELISA Assays. Antibodies were adapted to immunohistochemistry (IHC) by Avrameas and to enzyme-linked immunosorbent assays (ELISA) by Engwall and Van Weemen. At this time, antibodies were isolated from the serum of immunized animals and resulted in a polyclonal solution of antibodies. These antibodies were labeled with enzymes, instead of radioactive particles that were previously used, including the horseradish peroxidase (HRP) enzyme and the alkaline phosphatase (ALP), to be easily detected.
- 1971: Fluorescent Antibodies for Flow Cytometry. The first flow cytometer was built in 1965 but we had to wait until 1971 to see fluorescent Ab usage adapted to this technology. For the first time, Julius et al. sorted B cells with a flow cytometer by labeling them with a fluorescent Ab. Shortly after in 1975, Gratzner et al. use a fluorescent Ab for the measurement of cell proliferation. Gratzner developed a polyclonal Ab and then a monoclonal in 1982 recognizing bromodeoxyuridine, an analog of the thymidine, and used a secondary Ab labeled with fluorescein.
- 1975: First Hybridoma. Georges Köhler and César Milstein generated the first immortal hybridoma, fusing myeloma cells with B cells, that secretes Ab with a single specificity against sheep red blood cells.
- 1981: First Western Blot. The Southern blot technique was adapted to proteins and became known as the western blot, where proteins migrate by electrophoresis in polyacrylamide gels and are transferred to a membrane for detection by specific antibodies.
- 1984: First Chromatin Immunoprecipitation. Lis and Gilmour developed the chromatin immunoprecipitation (ChIP) assay to analyze the RNA Pol II/DNA interaction in Escherichia coli.
- 1984: First Recombinant/Chimeric Antibody. Morrison SL. and Neuberger MS. independently developed the first chimeric antibodies. The immunoglobulin gene from a mouse hybridoma cell line was cloned into a vector to be manipulated. Morrison et al. replaced the mouse Fc fragment with a human Fc fragment. Neuberger et al. expressed the plasmid in a non-myeloma cell line, J558L (plasmacytoma cells).
In less than a century, antibodies went from being unknown proteins to becoming indispensable for research and are now widely used for therapy.
Polyclonal Vs. Monoclonal Antibodies

When an external component such as a virus or bacteria enters a body, macrophages and dendritic cells capture it, digest it, and present the different antigens to the B cells. After antigen presentation, B cells undergo a process called somatic hypermutation to generate antibodies that are specific to these antigens. During this process, the variable regions of the antibody genes are adapted to contain a unique antigen-binding site.
One B cell produces one specific antibody that binds to one epitope of the antigen. Serum of immunized or infected animals will contain many different antibodies, referred to as polyclonal antibodies, produced by many different B cell clones. The individual B cell clones can be isolated to generate monoclonal antibodies. Monoclonals and polyclonals both display positive and negative properties and the best antibody for each use must be wisely chosen depending on the application.
Polyclonal Antibodies
The first antibodies used in research came from full serum containing polyclonal Abs. Polyclonal antibodies are generated by different B cells displaying different sequences of the variable regions of the Ab genes, and thus recognize different epitopes on the same antigen. Polyclonal antibodies for research use are most commonly produced in rabbits, but they can come from mice, horses, sheep, goats, and other animals.
To generate polyclonal antibodies, animals are injected with the antigen of interest to induce a primary immunological response. Then, they are usually subjected to a second and a third antigen injection to increase the Ab titer in the serum. After immunization, the serum can be directly used, or polyclonal Abs can be purified to obtain a solution free from other serum proteins.
The choice of which animal to use to generate polyclonal antibodies depends on the amount of Ab needed, the immune response (phylogenetically distant animals the antigen donor will have a stronger immune response), and the Ab characteristic (isotype, class). Horses and goats are usually used when a large quantity of antisera is necessary. Chicken is phylogenetically distant from mammals like humans and mice, so their immune response can be stronger. Moreover, in chickens, immunoglobulins are transferred into the egg yolk, and therefore harvesting Abs from eggs eliminates the need for the invasive bleeding procedure.
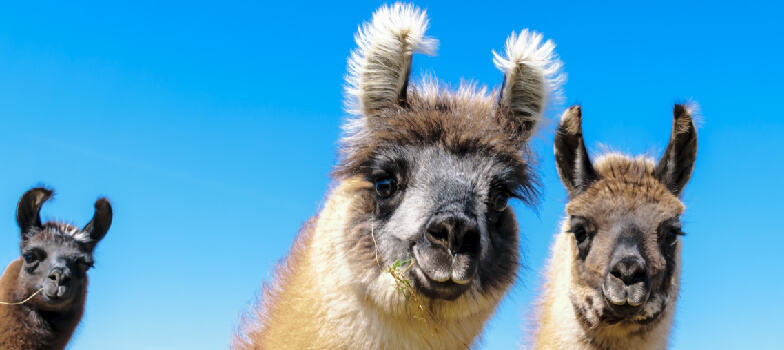
Some unconventional animals such as llamas can also be exploited for antibody production. The llamas, like every camelid family member, can produce antibodies with an original scaffold made of only heavy chains (IgG2 and IgG3). The camelid antibodies have several desirable properies, such as stability as solubility, and their smaller size provides some benefits for manufacturing.
The advantages of polyclonal antibodies are that they can recognize several epitopes of the antigen and are generally less expensive to produce. They are particularly useful for immunoprecipitation experiments including co-IP and ChIP assays. However, polyclonal antibodies that are from whole sera can contain non-specific Ig molecules. Since each lot of polyclonal antibodies usually comes from a different animal, they can display weak reproducibility. Moreover, the animal bleeding necessary to retrieve the serum creates ethical questions regarding animal experiments.
Monoclonal Antibodies
Monoclonal antibodies are generated from one single B cell clone and display only one sequence in the variable region of the Immunoglobulin and bind to only one epitope. This was made possible thanks to Georges Köhler and César Milstein who developed the first hybridoma in 1975.
Monoclonal antibodies were initially products in mice. After immunization, mouse B cell clones are fused to myeloma cells and become immortal. The hybridomas are then screened and tested for specificity. The specific hybridomas can be cloned and expanded to increase antibody production. Mice are still widely used for monoclonal production, but monoclonal antibodies are also produced from rabbits or other mammals.
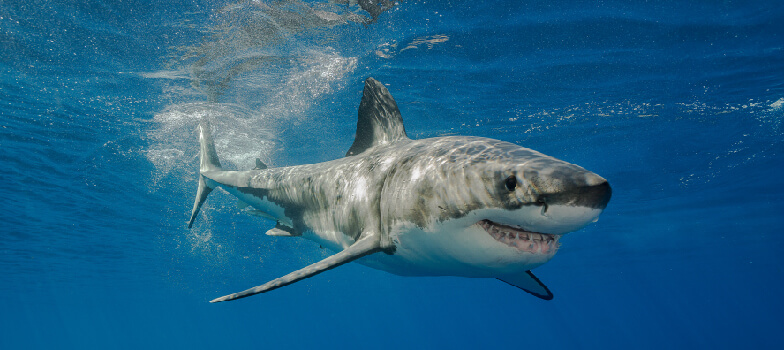
Another interesting and surprising species used for monoclonal antibody production is the shark. Shark blood contains a high level of urea that helps them survive in the marine environment, making their Ab structure much more robust than that of other vertebrates. Sharks produce a specific type of Ig, the IgNAR, with stability and penetrability properties much higher than other Ig isotypes.
As compared to polyclonals, large-scale production of monoclonal antibodies doesn’t need animals and the specificity and reproducibility from one batch to another is better. Therefore, monoclonal antibodies are the ones used for immunotherapies to avoid patient rejection and off-target effects. However, monoclonal antibody development can be more expensive.
How are Monoclonal Antibodies Made?
The original idea that led to the invention of monoclonal antibodies was to be able to continuously produce antibodies in vitro to improve antibody consistency and availability. Georges Köhler and César Milstein had the idea to fuse the splenocytes from the immunized mice to mouse myeloma cells, creating hybridoma cells. Myeloma cells are known to produce antibodies and they are immortal – meaning that they can divide indefinitely and the hybridomas therefore can be a sustainable and scalable source of antibodies.
After 5 months of culture, the hybridomas didn’t accumulate mutations at a significant rate, suggesting that these cells could grow forever and produce the same antibodies.
Since this first hybridoma publication in 1975, the method has been improved but the general technique remains the same.
Main Steps in Generating Monoclonal Antibodies
The main steps to follow to produce monoclonal antibodies are outlined briefly below.
- Animal immunization (mice, rabbits, sharks…).
- Splenocyte collection from the immunized animal.
- In parallel, immortal myeloma cells carrying a deficiency in the nucleotide salvage pathway, either thymidine kinase (TK) or hypoxanthin-guanine phosphoribosyltransferase (HGPRT), are cultured. This allows selection for fused cells in subsequent steps.
- B cells from the immunized animal and the myeloma cells are fused.
- B cell-myloma hybrids (hybridomas) are selected in HAT media (containing hypoxanthinem, aminopterin, and thymidine). Aminopterin prevents de novo nucleotides synthesis. Non-fused myeloma cells defective in the nucleotide salvage pathway cannot replicate under these conditions. Non-fused B-cells can multiply but are not immortal, so they will die after a few replication cycles. Only fused hybridomas can replicate indefinitely and de novo synthesize nucleotides, and thus survive in this selective medium.
- Single-cell cloning of hybridoma: cells are dispersed into microplates, usually by limiting dilution or cell sorting to achieve clonality. This stage may require multiple rounds to ensure genomic stability since chromosome eviction is a common occurance.
- Supernatant from hybridomas containing the monoclonal antibodies is screened to test antibody specificity and sensitivity.
- The chosen hybridomas are expanded to scale up antibody production.
- Desirable antibodies are harvested and purified for use in experiments.
Crucial Points to Consider When Generating Monoclonal Antibodies
To produce a suitable monoclonal antibody, several critical points must be considered: the cell line, the culture medium, the culture conditions, the production platform, and the production system.
The cell line should be stable and produce/secrete the monoclonal antibodies at a high level and in the right conformation. Mammalian cells are used most commonly, but microorganisms such as yeast or plants including maize, soybean, and tobacco can also be used.
For best results, the culture medium composition used with hybridoma cells should be maintained from one lot to another. To achieve the best consistency between lots, it is preferable to avoid using animal-derived products, such as serum, in cells used for monoclonal antibody production.
The culture conditions display two phases, one where the conditions promote cell division and another that promotes antibody production. Once the appropriate cell density is achieved, the culture conditions are shifted to promote Ab production.
Regarding the production platform, there are 3 solutions: batch, continuous fermentation, and fed-batch process (the most common at industrial scale). In batch, the medium is not renewed during the production so with time, nutrients decrease, and the cells stop producing Abs. During continuous fermentation, the medium is continuously renewed to maintain the right concentration of growth factors. In the fed-batch process, nutrients are added at specific intervals. The manufacturer can switch from the growth process to the production process and vice versa quite easily.
The production system used will be dependent on the desired final product. Production of antibodies that are designated “for research use only” generally does not necessitate high-scale production. However, for monoclonal antibody production for applications like immunotherapy, the required quantity and quality are much higher. There are different types of bioreactors that can be used to scale up monoclonal antibody production, such as air-lift bioreactors, stainless steel stirred tank bioreactors, disposable wave bioreactors, and roller bottles – each with their own set of benefits and limitations.
How Phage Display Libraries Improve Monoclonal Antibody Production
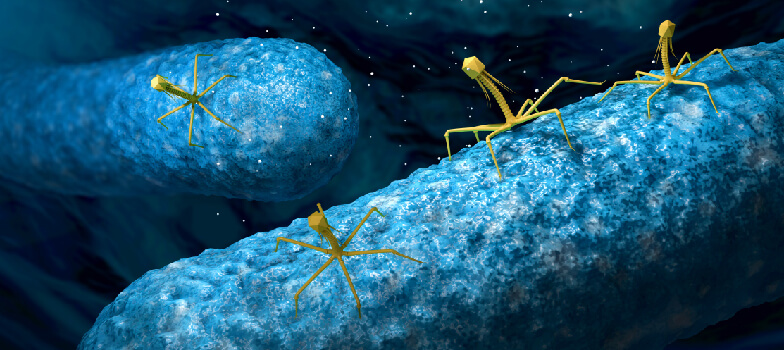
A major improvement of the monoclonal antibody generation protocol was the creation of phage display libraries. Bacteriophages, or phages for short, are a family of viruses that infect bacteria. With phage display libraries, a library of Fab regions (variable region of the Ig) is cloned into a bacteriophage plasmid vector. The Fab-modified bacteriophage vectors infect bacteria and produce the virus particles with capsid proteins containing viral proteins and the Fab fragment. Once the library is complete, the affinity between the different Fab regions in the library can be screened for immunogenicity, and the cell transformed with the plasmid that contains those genes can be sequenced.
There are several advantages offered by this methodology:
- A single phage library can generate a large number of new antibodies.
- There’s no requirement for animal immunization steps. It is an in vitro process by which a wide variety of antigens, including toxic antigens, can be tested.
- This method is fast. The desired monoclonal Abs are rapidly obtained.
Phage display technology was one of the first steps towards recombinant Ab technology expansion.
Recombinant Antibodies: The Next Generation of Antibody Technology
Polyclonal and monoclonal antibodies have revolutionized biomedical research through their use in different experimental applications. However, both monoclonal and polyclonal antibodies have limitations and weaknesses, leading many researchers to turn to recombinant antibodies.
Weaknesses of Monoclonal and Polyclonal Antibodies
Many publications have shown some discrepancies in the results of experiments using both monoclonal and polyclonal Abs. The reproducibility issues in the data were due to different parameters including batch-to-batch variation and non-specific antibody binding.
This kind of variation is understandable, and maybe even expected, for polyclonal Abs since they come from animal serum and every new batch is prepared from a different animal or a different immunization. However, unexpectedly, monoclonal Abs that are supposed to be monospecific can also display variability. Hybridomas have been shown to express additional functional variable regions. Bradbury et al. compared 185 hybridomas and demonstrated that 32% of them produced additional heavy and light chains leading to non-specific binding by the antibodies that were produced, decreased binding signal, and a decreased signal-to-noise ratio.
To try to overcome these problems, a new generation of Abs has been developed: the recombinant Abs.
What are Recombinant Antibodies?
Recombinant antibodies are a type of monoclonal antibodies that are generated in vitro from a synthetic gene without immunizing any animals or cultivating any hybridomas.
To understand the rise of recombinant antibody technology, let’s go back to 1984 when Morrison SL. and Neuberger MS. cloned the Ig genes from hybridomas to modify them in vitro and expressed the first chimeric Abs. Morrison exchanged the mouse Fc fragment with a human Fc fragment and Neuberger managed to produce Abs without hybridomas in plasmocyte cell lines.
What was called a “chimeric antibody” in 1984 was the first version of recombinant antibodies.
Recombinant Abs can be cloned from any species of antibody-producing animals. Once the sequence is cloned, it is possible to modify it – this is one of the major advantages of recombinant Abs. For example, Fc fragments from a species can be exchanged with another species, and the variable chain on the Fab fragment can be mutated to alter binding specificity or affinity. The above-mentioned phage display libraries are generated in this way and expressed in yeast or phage to be tested.
Recombinant Abs have several advantages as compared to monoclonals. The first is the increased reproducibility of the technology. The sequence of recombinant Ab gene is known and cloned, making them more reliable and more reproducible than monoclonals. The second advantage of recombinant antibodies over monoclonal antibodies is the time required to generate them. Whereas hybridomas need multiple months to produce effective Abs, recombinant Abs technology decreases the production time to just a few weeks in most cases. Finally, the generation and manufacturing of recombinant antibodies are animal-free technologies. Conditions and rules for animal experimentation are becoming more and more stringent around the world and the production process of recombinant Abs does not involve any animals.
The full control over the Ab gene sequence as well as the reduced turnaround time make the recombinant Abs useful tools for research as well as diagnostics and therapeutics.
How are Recombinant Antibodies Made?
The most common immunoglobulin isotypes, such as IgG, display a Y shape made up of 2 heavy chains and 2 light chains that are linked together by disulfide bonds. Each chain has 2 regions, one constant and the other variable. The variable region undergoes somatic mutation in B lymphocytes to be able to recognize the target antigen. To generate recombinant Abs, Ig genes must be cloned, either from existing hybridomas, or from immunized animals, from which either B cells or plasma cells can be used as source material, expressed in a biological system, and the obtained library must then must then be tested. Once a suitable sequence is found, a high-scale expression system can be used to produce large quantities of the recombinant antibody.
What Portions of the Antibody Gene Should be Cloned?
There are several different approaches to cloning genes for the generation of recombinant antibodies, including full-length IgG, only the Fab fragment, single-chain variable fragment (scFv), and single-domain fragments.
The full-length IgG approach is straightforward, both the heavy and light chains are cloned and expressed, generating full-length antibodies. This approach is desirable when the final product is complete antibody proteins that are similar to endogenous antibodies.
The Fab fragment is the antigen-detecting portion of the antibody obtained after Ig digestion with the enzyme papain. Cloning only the Fab region of antibody genes is useful when generating monovalent recombinant antibody proteins.
The scFv contains the variable region of the light chain and the heavy chain in a single-gene format connected with a peptide-linker and results in a functional antigen-binding site. Interestingly, scFvs can be used as drug candidates in their own right, as well as being vehicles for drug candidates. Their small size renders the scFv highly penetrative, with fast blood clearance.
Finally, single-domain antibody fragments, also known as nanobodies, are derived from camelid and shark Igs that contain only the variable region of the heavy chain. The main advantages as compared to scFv technology are better folding and stability characteristics due to the absence of a linker and their smaller size.
Library Generation for Antibody Display and Clone Selection
Recombinant antibody libraries require the used of expression systems that are highly competent (easy to transfect) and able to express, process, and secrete the recombinant Ab. For library generation, phage, yeast, and ribosome systems are commonly used. Mammalian cell systems such as CHO and HEK cells are also used routinely.
Phage display can screen small antibody fragments with a large antibody library. Ribosome display has an even bigger library capability, up to 1014 unique sequences, without limitations of transformation efficiency and acquired mutant antibody library since no cell system is involved. Yeast display is the best non-human system for human antibody expression because the yeast expression system is similar to mammalian cells.
Once the library has been generated, the selection process can begin. The antigens of interests are coated in a microplate and the antibody library is added. If the recombinant Ab binds the target antigens, the clone will stay in the well after washing and the unspecific Abs will be washed away. These selection steps are repeated 2-3 additional times to retrieve the clone producing the most specific Abs that bind the antigen with the highest affinity. Increasing stringency of binding conditions can select for stronger binders.
Large-Scale Production of Recombinant Antibodies
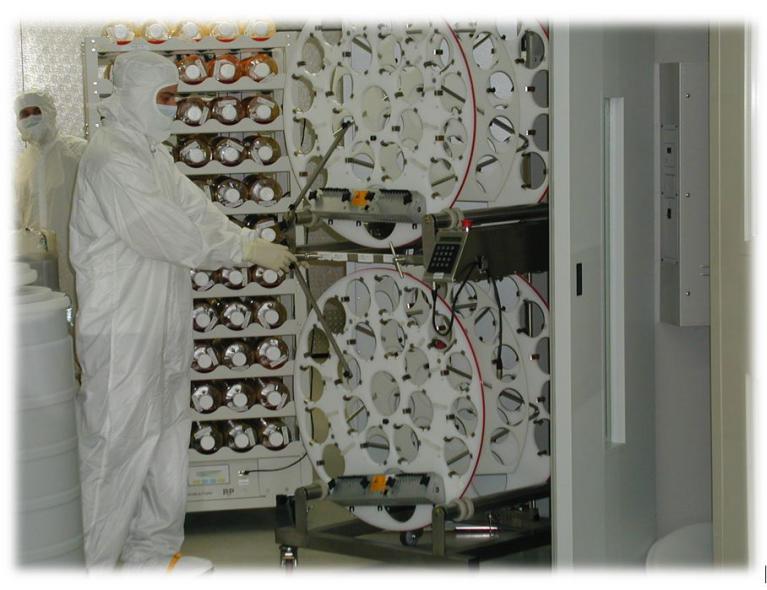
Once the best Ab sequence is identified, this clone can be amplified to produce large amounts. The best performing phage display clones can either be propagated in E. coli or yeast or re-introduced back into mammalian systems for production.
The expression systems are generally easy cell systems to scale up. Similar to the large-scale production of monoclonal Abs, the culture medium for recombinant antibodies has to be tightly controlled to prevent Ab secretion arrest. The purification step is also crucial especially if the Ab is going to be used in the clinic. Specific chromatography resins can be used to ameliorate binding characteristics and elution profiles. Moreover, the Ab sequence can be engineered to express a tag to help the purification process.
AbFlex® Recombinant Antibodies
Active Motif is a leading manufacturer and provider of antibodies for epigenetics and gene regulation studies. We have developed a proprietary platform to convert monoclonal antibodies into recombinant AbFlex antibodies. The AbFlex Abs are full-length antibodies containing both the heavy and the light chains. Most of the AbFlex antibodies available from Active Motif were derived from hybridomas expressing well-characterized Abs or directly cloned from single B cells or plasma cells. The antibody genes from these hybridomas have been sequenced and cloned to be expressed as a recombinant Ab. To make these recombinant Abs even more useful for the scientific community, Active Motif modified the antibody structure to include a unique design containing functional sequences useful for a variety of antibody applications.
AbFlex Antibody Structure
More specifically, AbFlex recombinant antibodies are full-length IgG2a mouse antibodies with cloned variable regions of interest from human, mouse, rabbit, or rat origin and are expressed with 3 different tags at the heavy chain C-terminus. The molecular tags on AbFlex antibodies include a Sortase A5 recognition site, a Biotinylation Tag, and a 6xHis Tag.
Using Sortase to Label AbFlex Antibodies
Sortase A5 is a transpeptidase (protein ligase) derived from Staphylococcus aureus that contains engineered sequence variants to make it 100-fold more active than the original sortase enzyme. Sortase A5 recognizes the LPXTG amino acid sequence, where X is any amino acid, cuts between the threonine and glycine residues, and covalently attaches chemical or biological probes containing a stretch of glycines.
Active Motif has developed multiple different Sortag-IT™ Labeling Kits to add fluorophores, HRP, or biotin in a site-specific manner to AbFlex antibodies. Labeling primary Abs directly with fluorophores for flow cytometry/IF or with HRP for western blot/immunocytochemistry avoids requiring the use of a secondary Ab, thus reducing the time of experiments as well as non-specific signal.
Non-specific labeling of antibodies is already routinely performed for other antibodies, so what is the main advantage of the specific sortase labeling process? Sortase activity enables the labeling to happen directly on the sortase recognition site so it is specific, and the labeling occurs stoichiometrically so a known amount of label can be added. The labeling site and the quantity of tag are fully controlled by the Sortase A5 enzyme.
Therefore, it is virtually impossible to over-label antibodies or to block antibody binding by masking the AbFlex variable region and interfere with antigen/Ab recognition. Additionally, during the Sortase A5 reaction, the other tags on AbFlex antibodies (Biotinylation Tag, 6xHis tag) are removed.
The Sortag-IT Labeling Kits contain everything you need to label AbFlex recombinant antibodies, from the sortase reaction to the stop solution and purification recommendations. However, if you want to label the AbFlex with your favorite conjugate, including nucleic acids and small molecules, Sortase A5 is available separately.
Biotinylation Tag & 6xHis Tag on AbFlex Recombinant Antibodies
The Biotinylation Tag is a 15 amino acid sequence that is recognized and biotinylated by the prokaryotic enzyme BirA. Adding a BirA labeling Tag to AbFlex antibodies allows for site-directed labeling with biotin. As for the Sortase A5 reaction, the quantity and the binding site of the biotin are fully controlled. Two molecules of biotin are added, which prevents over-labeling and Ab binding-site masking.
Finally, the 6x histidine tag enables purification by nickel chromatography or antibody capture using an anti-His tag antibody.
What Makes AbFlex Recombinant Antibodies Better than Other Antibodies?
As mentioned earlier, when hybridomas that produce monoclonal antibodies were meticulously analyzed, they were found to produce additional heavy and light chains in 32% of the cases. Therefore, the antibodies generated by hybridoma cells that contain the genes to express additional heavy or light chains are not actually monoclonal.
When monoclonal antibodies from hybridomas are converted to become an AbFlex recombinant antibody, they are tested in different applications to ensure the properties and characteristics of the recombinant Ab is as good as the parent monoclonal Ab. In many cases, the affinity and specificity of the recombinant version of the antibody improve because the AbFlex Abs are truly clonal.
Another benefit of using AbFlex antibodies is that because of their labeling capacity, secondary Abs are often no longer needed. Moreover, in experiments using fluorophores, such as flow cytometry or IF, more colors can be used without regard to Ig species and fear of the species cross-reactivity that are problems when using secondary antibodies.
Furthermore, Active Motif developed a special expression system for the AbFlex antibodies, giving them high batch-to-batch reproducibility and high Ig secretion level. The DNA sequences used are are validated for each lot, eliminating the possibility of drifts or variations in the sequence.
Active Motif’s offering of AbFlex recombinant antibodies is primarily focused on histones/histone post-translational modifications, transcription factors, and other epigenetic proteins. However, we also offer a custom AbFlex generation service to convert monoclonal antibodies from any hybridoma cell line into AbFlex recombinant antibodies. This service can also introduce additional tags or sequences into the recombinant antibody to offer additional flexibility and different applications can be tested to provide the most useful AbFlex antibody as possible.
What’s Next for Recombinant Antibodies?
Recombinant antibodies are a new tool to add to a researcher’s toolbox. With the multiple engineering possibilities that can be performed to alter and improve the properties of the antibodies, the potential applications and opportunities for recombinant antibodies are tremendous.
Regarding research applications, the development of more specific, sensitive, and reproducible antibodies is an improvement that will lead to increased reliability and reproducibility of scientific results. For current antibody-based applications such as western blot, IHC, IF, and flow cytometry, the direct labeling of the primary Abs will increase the sensitivity of the signal and the specificity. Moreover, the Ig type and species are not a limitation anymore for experiments with multiple targets (e.g. IF and flow cytometry). Engineering could also modify the Ab structure to improve in vivo imaging with bioluminescence signal or clinical trials in animals.
The fast screening method of display library technology to identify the best antibody/antigen combination will facilitate the development of Abs specific for newly discovered proteins and thus accelerate research in general.
Large-scale production of recombinant Abs is less expensive than other methods for generating polyclonal or monoclonal antibodies, rendering this kind of product more affordable for academic budgets, bigger experiments, as well as applications requiring a higher amount of Abs (ChIP, IP).
Looking at the bigger picture, recombinant Abs are also transferable for in vitro diagnostic and clinical uses. Quality controls are much more stringent for these kinds of applications and investment in time and money for validation is needed. However, the intrinsic qualities of recombinant antibodies, such as reproducibility, sensitivity, and easy screening, make them perfect candidates for diagnostics and clinical use.
Until now, Abs used for immunotherapy and diagnostics have been monoclonal Abs (e.g. Tocilizumab, Certolizumab). Converting these Abs into recombinant versions, despite the additional tests that may have to be performed initially, could make antibody-based therapy more affordable and shorten the development time. Engineering could also make recombinant Abs easier to penetrate some tissue or adapt them for use as a carrier to help target a drug to the cells/tissues of interest.
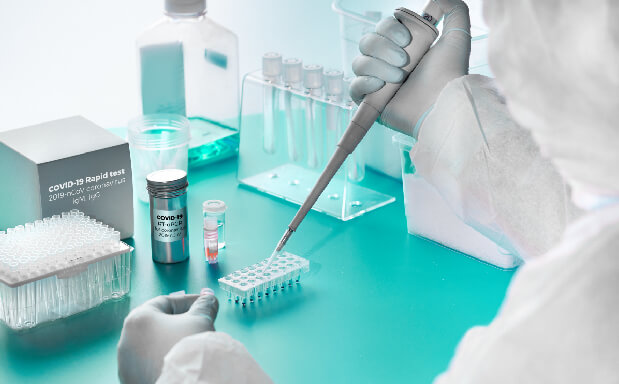
A great example of the benefits of development speed and reproducibility of recombinant antibodies comes from a collaboration between scientists at Active Motif Shanghai (a subsidiary of Active Motif, Inc.), Fudan University, and its affiliated Public Health Clinical Center to isolate, clone, express, purify, and characterize antibodies from patients infected with the COVID-19 virus. The team of researchers used Active Motif’s proprietary AbEpic™ platform to develop and characterize 9 recombinant human Abs against the SARS-Cov-2 spike protein within just a few weeks. These antibodies are currently being further developed and validated by other researchers for use in diagnostic assays.
Summary: Recombinant Antibodies Represent Both the Present and the Future
The history of antibodies being used for research or in clinical settings showed the ingenuity of scientists for twisting a biological process like immunity for their purposes. From the use of total serum to the development of recombinant Abs, antibody-based technologies have come a long way.
Polyclonal antibodies are easy and relatively inexpensive to produce, and their poly-binding capacities make them suitable for recognizing the 3-D conformation of proteins. However, polyclonals demonstrate an increased risk of non-specific binding, require immunizing animals, and they have serious issues with lot-to-lot variability.
Monoclonal antibodies are more expensive to produce but are renewable and therefore better suited for clinical and diagnostic applications.
Polyclonal and monoclonal antibodies will still be used for a long time for various applications based on their long history of successful use before the recombinant Abs obtain the same level of characterization and recognition by the scientific community.
It is clear that recombinant Abs are promising tools and that they have bright futures in both research and clinical use. The advantages that recombinant antibodies offer, such as full control over their production, from Ab sequence to Ab/antigen binding, the possibilities of engineering, and the absence of animal use, render the recombinant Abs very attractive for research, diagnostic, and clinical applications.
Several commercial antibody providers, with Active Motif leading the way, have already started to convert their hybridoma-generated monoclonal antibodies into recombinant Abs, and more and more recombinant Abs are being used in scientific publications.
Recombinant antibody technology is full of promises. The short development time and the ability to easily scale up the production will allow scientists to answer to more intricate and complicated biological questions and thus lead to better disease understanding and new therapeutic strategies.
About the author

Anne-Sophie Ay-Berthomieu, Ph.D.
Anne-Sophie was born in the south of France and grew up between the Mediterranean Sea and the Pyrenean Mountains. She grew up as a science fiction fan, leading her to specialize in molecular biology and genetics during graduate school at the University of Lyon, France (secretly hoping her research would give her superpowers!). After living in different places for work, she is back in Lyon, France where she shares her time between her husband, her family, and her friends. During her free time, Anne-Sophie challenges herself with hiking, climbing, racing, and traveling in foreign countries – while waiting for her superpowers to grow!
Contact Anne-Sophie on LinkedIn with any questions, or to tell her about your superpowers.
Related Articles
Guide to Generating the Best ChIP Data
March 15, 2019
The chromatin immunoprecipitation (ChIP) assay has become one of the most popular laboratory techniques to investigate the association of DNA-binding proteins and histones with chromatin. This article covers the major challenges of ChIP assays and how to overcome them to generate the best ChIP data.
Read More
Complete Guide to Sonication of Chromatin for ChIP Assays
January 31, 2020
Chromatin shearing/sonication is one of the most important steps in the ChIP workflow and is generally the step that exhibits the most variability. This article discusses the differences between each available sonication method and highlights their pros and cons.
Read More
<< Back to MOTIFvations Blog Home Page